As global renewable energy systems rapidly expand, lithium iron phosphate (LFP) batteries have become a cornerstone of grid-scale energy storage due to their stability and cost-effectiveness. However, thermal runaway (TR) remains a critical safety challenge, capable of triggering fires and explosions. This study evaluates multi-parameter sensor configurations for early TR detection in LFP battery compartments, while exploring synergies with Maximum Power Point Tracking (MPPT) systems to enhance energy efficiency and safety.
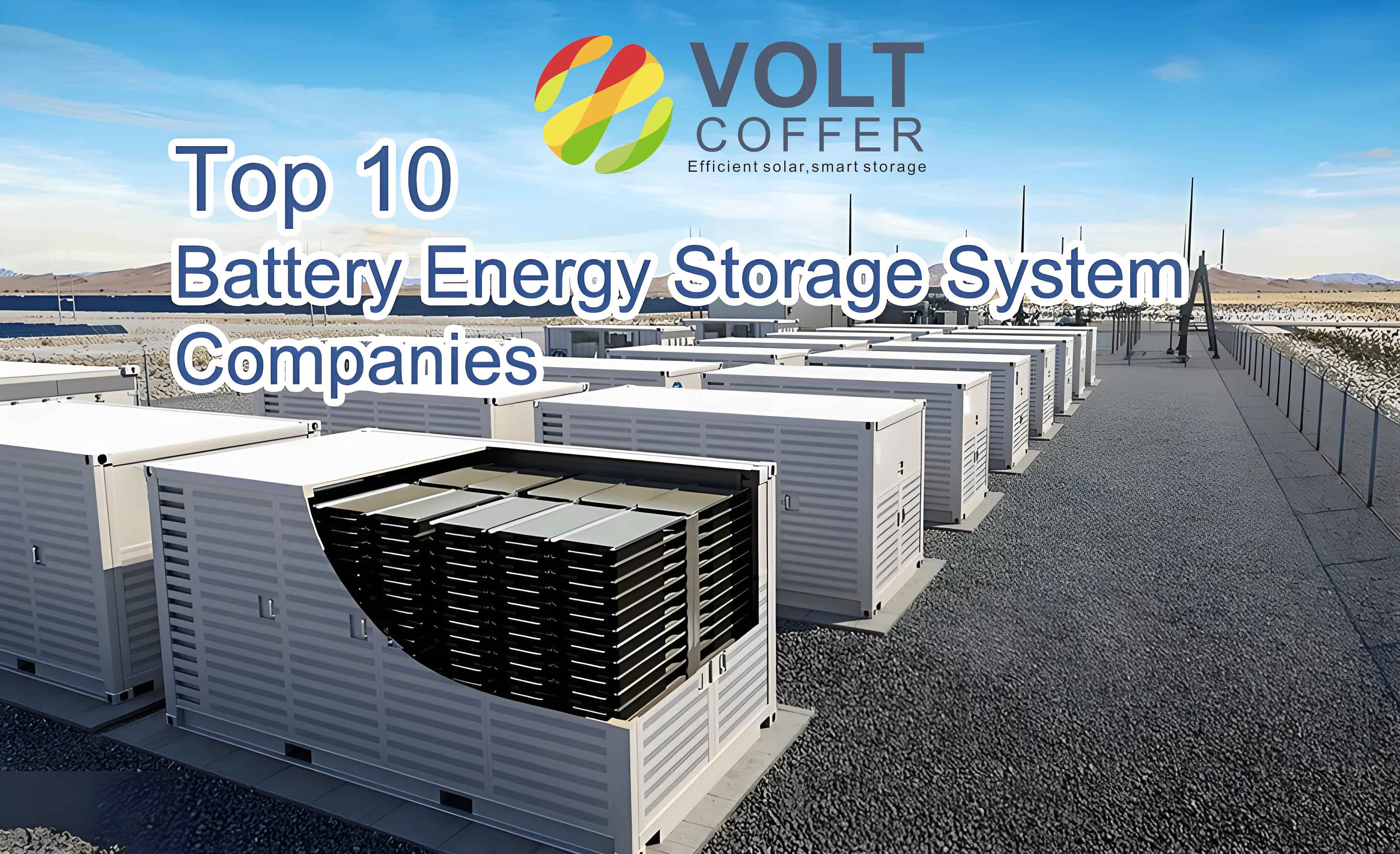
1. Experimental Framework and Sensor Performance
A custom 40-foot energy storage compartment was equipped with five composite sensor arrays (A–E), each integrating distinct detection principles for H₂, CO, CO₂, volatile organic compounds (VOC), smoke, pressure, and temperature (Table 1).
Table 1: Sensor configurations and detection principles
Sensor | H₂ Detection | VOC Detection | CO Detection | CO₂ Detection | Smoke | Temp. | Pressure |
---|---|---|---|---|---|---|---|
A | Electrochemical | Solid Polymer | Electrochemical | – | Semiconductor | Thermocouple | – |
B | Semiconductor | Solid Polymer | Electrochemical | – | Semiconductor | Thermocouple | – |
C | Semiconductor | Solid Polymer | Electrochemical | – | Semiconductor | Thermocouple | Piezoresistive |
D | Catalytic Combustion | Photoionization | Electrochemical | IR Optical | Semiconductor | Thermocouple | – |
E | Semiconductor | Solid Polymer | Electrochemical | IR Optical | Semiconductor | Thermocouple | – |
Key findings:
- Catalytic combustion H₂ sensors detected TR 100–300 s faster than electrochemical/semiconductor variants.
- Photoionization VOC sensors outperformed solid polymer types by 600 s, detecting electrolyte leakage and blue film decomposition.
- VOC signals emerged first (pre-TR phase), followed by H₂, CO, and smoke (TR onset), with CO₂ lagging due to delayed SEI decomposition.
2. Thermal Runaway Dynamics and MPPT Synergies
TR was induced via 950W heating, with/without ignition. Critical parameters were analyzed to establish detection thresholds and propagation rates.
2.1 Gas and Smoke Propagation
The compartment’s airflow dynamics followed buoyancy-driven plume rise and ceiling jet dispersion (Figure 1). Propagation rates (v) were calculated using:v=∣tn,ε−tn+1,ε∣2400(n=1,2,3,4)
where tn,ε = detection time at sensor n for threshold ε.
Table 2: Propagation rates (mm/s) under different conditions
Parameter | Edge Heating (No Ignition) | Center Heating (No Ignition) | Center Heating (Ignition) |
---|---|---|---|
H₂ | 28.26 | 23.48 | 97.61 |
CO | 29.57 | 27.21 | 132.76 |
VOC | 21.30 | 15.41 | 132.53 |
Smoke | 26.15 | 21.75 | 129.95 |
CO₂ | 51.90 | 35.71 | 194.80 |
Ignition accelerated propagation 4–8× by introducing thermal buoyancy and combustion-driven convection. Notably, MPPT-integrated systems could optimize ventilation responses by aligning airflow control with real-time power demands, though this requires further study.
2.2 Impact of Ignition on Detection
Ignition drastically altered gas composition (Table 3):
- CO, smoke, and CO₂ surged due to combustion.
- H₂ and VOC declined as electrolytes burned.
- Ceiling temperatures rose sharply (0.78∘C/min vs. 0.10∘C/min without ignition).
Table 3: Parameter thresholds under ignition vs. non-ignition
Parameter | Non-Ignition Threshold | Ignition Threshold |
---|---|---|
H₂ (ppm) | ≥2000 | ≥2000 |
CO (ppm) | 684 | ≥1000 |
VOC (ppm) | ≥10,000 | 5778 |
Smoke (mg/m³) | 4801 | ≥10,000 |
CO₂ (ppm) | 1296 | ≥5000 |
MPPT algorithms could enhance safety by modulating charge/discharge rates during TR precursors, reducing thermal stress.
3. Sensor Placement and MPPT-Driven Optimization
Edge-mounted sensors detected TR 15–30% faster than center placements due to reduced airflow resistance. Recommended detector spacing:
- Edge zones: 0.64–1.28 m
- Central zones: 0.92–1.85 m
Integrating MPPT with sensor networks could enable adaptive compartment partitioning, where TR risks trigger localized power redirection to minimize cascade failures. For example, MPPT controllers could reroute energy flow from TR-prone modules to stable ones, balancing efficiency and safety.
4. Detection Strategy and Future Directions
A multi-stage TR warning system is proposed:
- Stage I (VOC > 100 ppm): Early alert for electrolyte leakage.
- Stage II (H₂ > 500 ppm): Confirm TR initiation.
- Stage III (CO > 200 ppm + smoke > 1,000 mg/m³): Activate fire suppression.
Future work will explore:
- MPPT-based thermal management to delay TR onset.
- Machine learning models fusing sensor data with MPPT logs for predictive TR mitigation.
- Catalytic combustion H₂ and photoionization VOC sensors as industry standards.
5. Conclusion
This study validates catalytic combustion H₂ and photoionization VOC sensors as optimal for early TR detection in LFP systems. Ignition dramatically alters gas dynamics, necessitating adaptive thresholds. MPPT integration presents untapped potential for enhancing both energy efficiency and TR response protocols. By aligning sensor networks with MPPT-driven airflow and power distribution, future energy storage systems can achieve unprecedented safety and performance synergies.
Formula Appendix
- Fick’s Diffusion Law:
ρDABdxdYA+ρDBAdxdYB=0
- Temperature-Dependent Diffusion:
DAB∝θ03/2P0−1