Modern low-voltage distribution networks face significant voltage regulation challenges due to the rapid integration of distributed photovoltaic (PV) systems. This study proposes a group-coordinated voltage control strategy that leverages the complementary advantages of PV inverters and energy storage inverters. By combining consensus algorithms with voltage-cost sensitivity analysis, the strategy optimizes reactive power utilization from PV inverters and active power dispatch from energy storage systems (ESS) to suppress voltage violations efficiently.
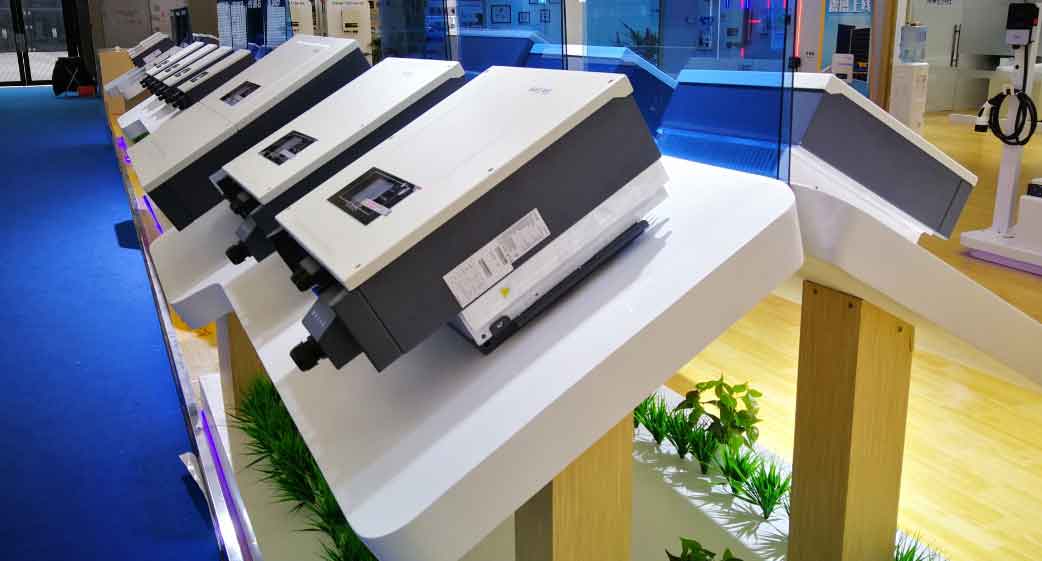
1. Voltage-Cost Sensitivity Framework
Voltage regulation effectiveness depends on the sensitivity of nodal voltage to power adjustments and the associated economic costs. For a node i, the voltage deviation ΔUi caused by power changes at node j is expressed as:ΔUi=Sij[ΔPjΔQj]=[SijU−PSijU−Q][ΔPjΔQj]
where SijU−P and SijU−Q represent the voltage-active and voltage-reactive sensitivity matrices, respectively.
The voltage-cost sensitivity factor (VCSF) quantifies the economic efficiency of voltage regulation devices:FijU−C=CjΔUij
For PV inverters and energy storage inverters, this factor becomes:FPV,ijU−C=cPVSijU−Q,FESS,ijU−C=cESSSijU−P
where cPV (≈0.067 USD/kvar·h) and cESS (≈0.6–1.0 USD/kW) denote the unit regulation costs.
Key Insight:
- FPV,ijU−C>FESS,ijU−C in low-voltage networks (due to high R/X), favoring PV inverters for reactive power regulation.
- Downstream nodes exhibit higher VCSF than upstream nodes, prioritizing localized control.
2. Group-Coordinated Control Architecture
The proposed strategy divides PV and energy storage inverters into voltage control groups based on their VCSF rankings.
2.1 Group Formation and Prioritization
- Group 1 (GV1): Nodes with moderate VCSF (e.g., upstream PV/ESS).
- Group 2 (GV2): Nodes with high VCSF (e.g., downstream PV/ESS).
Control Workflow:
- PV Inverter Stage: GV2 deploys reactive power first; GV1 supplements if GV2 reaches capacity.
- Energy Storage Inverter Stage: GV2 discharges/charges active power; GV1 intervenes if GV2 constraints are met.
2.2 Consensus Algorithm Design
- PV Inverters: Consensus variable = reactive power utilization (μ):
μGVi,j(k+1)=m=1∑NPβGVi,jmPVμGVi,m(k)+dGVi,jPVλ1(μGVi,j(k)−μGVi,nref(k))
- Energy Storage Inverters: Consensus variable = SOC change (ΔS):
ΔSGVi,j(k+1)=m=1∑NbβGVi,jmESSΔSGVi,m(k)+dGVi,jESSλ2(ΔSGVi,j(k)−ΔSGVi,nref(k))
Parameters λ1=0.35 and λ2=0.40 balance convergence speed and accuracy.
3. Case Study: IEEE 14-Node Low-Voltage Network
3.1 Simulation Setup
- PV/ESS Configuration:
Node3,4,7,9,13,145,8PV Capacity (kVA)1012ESS Capacity (kWh)810ESS Power Rating (kW)1.62.0
- Voltage Limits: Umin=0.95pu,Umax=1.05pu.
3.2 Performance Comparison
Three strategies were evaluated:
- S1: PV inverters only.
- S2: Energy storage inverters only.
- S3: Proposed group-coordinated control.
Voltage Regulation Outcomes:
- S1 failed to eliminate violations due to reactive power saturation.
- S2 required excessive ESS capacity (50.81 kWh) but achieved compliance.
- S3 reduced ESS usage to 8.03 kWh (15.8% of S2) by prioritizing PV inverters.
Economic Analysis:MetricPV Reactive CostESS Active CostTotal CostS2 (USD)0.0030.4930.49S3 (USD)5.854.8210.67
S3 lowered costs by 65% compared to S2.
4. Conclusion
The group-coordinated strategy demonstrates superior performance in low-voltage networks:
- Reduced ESS Dependency: Energy storage inverters are activated only when PV capacity is exhausted.
- Cost Efficiency: Prioritizing high-VCSF groups minimizes reactive/active power waste.
- Scalability: Consensus algorithms enable plug-and-play integration of new PV/ESS units.
Future work will explore dynamic group reconfiguration and real-time VCSF adaptation.